Farming for the future: Using technology to adapt agriculture for a changed world
By Christian Yonkers
Most of the world utilizes a “linear” pathway of agricultural production. This “take-make-use-waste-pollute-repeat” approach is unsuitable for scaling circular and sustainable agriculture systems needed for a warming climate. Linear systems are dependent on external inputs like trucked-in fertilizer, pesticides, and feed, most of which come from hundreds or even thousands of miles off-farm. According to an Ellen MacArthur Foundation report, less than 2% of agricultural food waste is reintroduced back into agriculture systems. The remaining 98% enters the environment as pollutants or ends up in landfills.
The main goal of a circular agricultural system is to fuse economic growth with sustainable resource use through regenerating natural resource systems, keeping resources within cycles as long as possible, and eliminating waste and pollution from production and consumption. As grain production contributes disproportionately to agriculture’s environmental impacts, it should be a primary target for circular strategies.
Dr. Bruno Basso is a John Hannah Distinguished Professor of earth and environmental science at Michigan State University. Basso researches an ensemble of digital, mechanical, and biological technologies called “digital agriculture,” a suite of tools with significant promise in building and scaling circular agriculture systems for a changed world.
“Digital agriculture” for strategic fertilizer management and mitigation
Recent studies have opened a new frontier of data analysis enabling farmers to strategically apply fertilizer based on real-time field and climate data, providing less fertilizer waste and greater cost savings, pollution reduction, and higher crop yields. Integrating an ensemble of digital agriculture technologies, new plant genetics, and regenerative agriculture methods can greatly improve nutrient cycling efficiency and climate mitigation.
Basso’s work uses remote sensing and artificial intelligence to identify the unproductive parts of a field where farmers routinely over-apply fertilizer. Reducing fertilizer use through strategic application not only saves money and boosts yields, but can play an important role in decreasing agricultural greenhouse gas emissions.
Nitrogen fertilizer is a major player in environmental damage. In the U.S., farmers are pressured to increase productivity and crop yield by using fertilizer, often at levels much higher than crops need. The environmental costs of overfertilization are steep, leading to an excessive richness of nutrients in groundwater and to massive plant and animal die off. Overfertilization also produces dangerous nitrous oxide emissions, a greenhouse gas 300 times more potent than CO2. Globally, agriculture is responsible for 14% of all anthropogenic emissions, and half of those result from fertilizer application.

Fertilizer application assessment based on data from field equipment, processed with Crop Monitoring.
Digital agriculture cuts fertilizer application 36%, said Basso. When properly implemented, this can reduce 300Kg CO2e per acre every season. This does not include emissions avoided from fossil fuel burning and fertilizer production and transport – only emissions directly saved from fertilizer emitting NO2 into the atmosphere.
For the 23 million acres of low-productivity U.S. cropland, this means a massive reduction of CO2e.
Basso and his team use satellite imagery to map nearly every acre of cropland in the United States. This baseline map can be supplemented with detailed information gathered from drones and aircrafts fitted with RGB, infrared, thermal, and lidar imaging devices used to determine chlorophyll levels, plant size and health, temperature, and more.
Suitability analysis determines within feet which areas respond well to fertilizer and other treatments, and prescribes targeted interventions to maximize profits and decrease environmental impacts of overfertilization. Taking yield maps from harvest, historical data, airborne imagery, and on-ground measurements, scientists are able to map a field’s historical ebbs and flows, and can plot these fluctuations with climate variability, change in farming practices, etc. The long term implications mean an expanded suite of tools for adapting to a changing climate, but the short term benefits of reduced fertilizer-related emissions and cost savings can’t be overstated.
Farmers implementing Basso’s prescription maps are paid $10 per acre on top of their cost savings from averted fertilization. Crops receiving targeted fertilizer treatment are placed beside ones receiving regular fertilization, showing that crops receiving less fertilizer on the same unproductive ground perform no better than those receiving more.
Electrification and automation
Robotics and other fully autonomous vehicles are co-emerging with electric vehicles, and will play a critical role in digital agriculture, said Basso. Small autonomous robots can help plant cover crops while a crop is still standing in the field. Weeds can be mechanically cultivated instead of spraying herbicide. If a field is too wet or crops too tender for large machinery to operate, small robots can weed, plant, and fertilize with minimal disturbance or soil compaction. Autonomous irrigation applies water directly to each row, saving water and delivering strategic irrigation exactly where it’s needed.
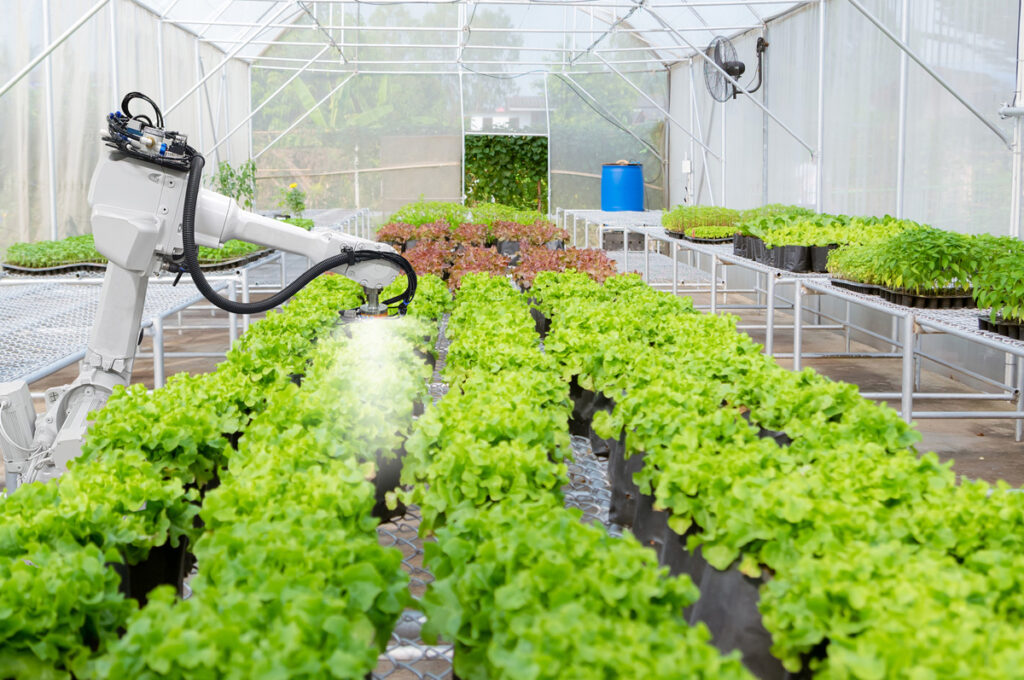
Small robots can weed, plant, and fertilize with minimal crop disturbance or soil compaction.
“It’s still too early,” Basso said about scaling robotics on the farm. “But it’s only a matter of time before they become perfect. They will be cheaper and they will do the job.”
Technological fixes are only a single piece to a very intricate puzzle, but they will help pave the way for an ancient yet fundamentally important facet of farming: Building soil organic carbon.
Farming carbon
Building soil organic carbon [ as opposed to soil organic carbon ] is the greatest form of agricultural adaptation, Basso said, and must be the chief aim of any mitigation or adaptation effort.
Soil organic carbon is a measureable component of soil organic matter. Organic matter makes up just 2–10% of most soil’s mass and has an important role in the physical, chemical and biological function of agricultural soils.
“If you want to adapt to increased climate variability, building topsoil, reducing erosion, allowing the return of carbon when the cover crop is killed, that is the way to do it,” Basso said.
Basso is working towards scaling the certification of emissions reductions associated with digital agriculture. He foresees a very near future where carbon is another cash crop like corn or soybeans, represented through actual carbon drawn down into the soil and the CO2e averted from fertilization and land use changes associated with conventional agriculture.
Part of Basso’s work focuses on including regenerative practices into his prescription maps. In poorly performing plots, Basso’s program first prescribes cutting fertilizer inputs. If needed, low yielding areas are removed from rotation and planted with nitrogen-fixing cover crops, native vegetation, and pollinator habitat. Farmers are paid to keep unproductive land out of crop rotation and implement restorative practices on them.
“Those areas will accumulate carbon over time, because you don’t put in fertilizer, you plant perennials,” Basso said.
Regenerative agriculture for adaptation
Technology and nature-based solutions should never be seen in isolation, but as integrated suites of tools, said Basso. No one tool is driving the solution itself, but it’s a piece of the puzzle.
“Adaptation and mitigation go together,” Basso said. “Regenerative agriculture is a specific category that helps in adapting, and digital agriculture does both. It helps you do strategic as well as tactical management to avoid the critical impacts of climate change.”
Digital agriculture builds efficiency through strategic planting, fertilizing, harvesting, and automation. Regenerative agriculture builds more natural resources.
“But they’re not mutually exclusive,” Basso said. “Digital ag allows you to manage regenerative fields much better.”
Technological and nature-based solutions are only part of the overall solution framework. Education and cultural acceptance are perhaps the greatest obstacles to greater adoption of digital agriculture. Moreover, farmers need support to weather the initial costs and learning curves associated with scaling digital agriculture.
Basso believes public-private partnerships should invest time, money, and resources into training and equipping farmers for the future interface of tech and ecosystems on the farm. These trusted institutions should be transparent about potential risks of changing farming practices, and provide support needed to make the transition.
“I think that farmers are not the ones who should always bear the risk when it comes to these things,” Basso said.
All of these tools are costly, but if history holds any lessons, they won’t be for long. In the span of a decade, smartphones have become a staple of modern life, and Basso believes the same is true for digital agriculture.
“The fact that farmer numbers are dwindling and farms are getting bigger, farmers need to embrace that technology will help them fight what they are already witnessing on their farms: Seriously increased climate variability,” he concluded.
Circular Economy for Food
By making great strides in productivity, the industrial food system has managed largely to meet the demands of a growing global population. However, this approach to food production, and the management of food by-products, is endangering biodiversity and human health. It has become clear that this food system is no longer fit for the 21st century and that a new model is required.
Soil carbon cycle through the microbial loop
Carbon dioxide in the atmosphere is fixed by plants (or autotrophic microorganisms) and added to soil through processes such as (1) root exudation of low-molecular weight simple carbon compounds, or deposition of leaf and root litter leading to accumulation of complex plant polysaccharides. (2) Through these processes, carbon is made bioavailable to the microbial metabolic “factory” and subsequently is either (3) respired to the atmosphere or (4) enters the stable carbon pool as microbial necromass. The exact balance of carbon efflux versus persistence is a function of several factors, including aboveground plant community composition and root exudate profiles, environmental variables, and collective microbial phenotypes (i.e., the metaphenome).